User:Shawndouglas/sandbox/sublevel33
2.1 Testing terminology
As you read through this chapter, you may discover terms like "polymerase chain reaction" (PCR) and "lateral flow assay" (LFA). If you're a laboratorian or have a clinical background, you may already be familiar with these terms. However, it seems prudent to at least briefly discuss a few of them before delving into coronavirus testing itself.
2.1.1. Introduction
Living organisms store information in their genetic material, using DNA or RNA as the information carrier. That information, or genetic code, essentially provides instructions for organism development, function, growth, and reproduction. In the late twentieth century, researchers were laying the groundwork for molecular diagnostics, the concept of examining an organism's genetic code and its associated biological markers to diagnose and treat disease on a more personalized basis. This requires an assay, an investigative procedure for assessing the presence of, or measuring the amount or functional activity of, a target analyte. In the case of molecular diagnostics, and more broadly molecular biology, the target is biological in nature, and thus biological assays are used. These biological assays are designed to accurately detect the presence of or enable counts of biological molecules, including DNA, RNA, proteins, cells, bacteria, and virus particles (e.g., viral plaque assays).[1]
2.1.2 Polymerase chain reaction (PCR)
Polymerase chain reaction or PCR is a molecular biology method that takes small amounts of DNA sequences and makes copies of (amplifies) them to the point of having enough material to sufficiently study or work with. The base technique can yield results in several hours and has a high level of sensitivity, with its ability to amplify the DNA to counts of millions or billions. PCR has been used in molecular diagnostics for testing prospective parents for being genetic carriers of particular diseases (i.e., expanded carrier screening)[2][3], tissue typing to ensure more effective organ transplants[4], and analyzing mutations in oncogenes to customize cancer treatments.[5] However, the method has also been applied to forensic science[6] and epidemiology.[7]
PCR and its variations have been used to characterize and detect infectious disease organisms such as human immunodeficiency virus (HIV), pathogenic tuberculosis bacteria, and Bordetella pertussis, which causes whooping cough.[8] Additionally, a selection of viruses can have their RNA detected using PCR, though the primers (short single-strand DNA fragments) used in the process must by sympathetic to the virus' genetic structure to ensure that only target virus material is amplified.[9] As it turns out, coronaviruses are RNA viruses, having some of the longest genomes of any RNA virus, and, detrimentally, the highest known frequency of recombination (the exchange of genetic material with another organism); this broadly means high rates of virus mutation, which interferes with maintaining consistent diagnostic detection and therapy.[10][11]
PCR comes in several variant methods. For example, while PCR monitors the amplification portion at the end of the overall process, real-time or "quantitative" PCR (qPCR) allows for the generation rate of the amplified product to be monitored at a particular point during each PCR cycle. Reverse transcription PCR (RT-PCR) is a combinatory process, applying reverse transcription (creating complementary double-stranded DNA [cDNA] from an RNA template) with PCR. If RT-PCR incorporates qPCR, you end up with "real-time RT-PCR" (rRT-PCR), sometimes referred to as "quantitative RT-PCR" (qRT-PCR). In the case of using PCR for detecting coronaviruses, more often than not we see some variation of RT-PCR, with or without real-time amplification monitoring. (It's important to not assume all RT-PCR processes incorporate real-time methods.)
How does PCR work in practice? The simplified version (see this JAMA Patient Page for a useful graphical explanation, using COVID-19 as an example) has a clinician obtaining a biological specimen from the appropriate location or source material. Then, special techniques are used to isolate viral (or in some cases, bacterial) genetic material from the specimen. (If RT-PCR is performed, the next step of reverse transcription of the isolated viral RNA into cDNA is also performed.) Once the viral genetic material is isolated, suitable primers that are sympathetic to the structure of the isolated genetic material are introduced. Those primers bind to the virus' genetic material and begin making copies of it. Fluorescent or other biomarkers that were attached to the copies during the PCR process eventually release from the copies, and an attempt is made to detect the presence of those biomarkers. The presence or absence of these markers drives the determination of a positive or negative detection for the sought-after virus.[12]
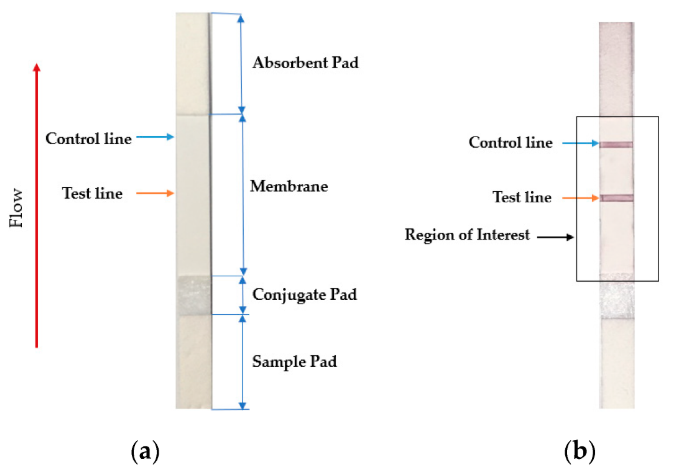
2.1.3 Lateral flow assay (LFA)
The lateral flow assay or LFA is another molecular biology method for detecting the presence of a target analyte in specimen material. In comparison to PCR, LFA has the advantages of being more rapid, low-cost, easy-to-use, and applicable at the point of care, though the average LFA is at best semi-quantitative in its results and has slightly lower sensitivity.[13][14] The method involves a cellulose-based strip with an ordered collection of specific "pads" and reagents that are reactive to a target analyte in a liquid, which is placed on the strip and moves across the various reagents using capillary and electrostatic interactions.[13][15][16] LFA has been used in molecular diagnostics for testing urine, saliva, sweat, serum, plasma, and other biological fluids for the presence of specific antigens and antibodies, as well as signs of gene amplification. The method has also been effectively applied to other industries outside healthcare, including the food and beverage, chemical, and environmental industries.[13]
In the realm of infectious disease, the LFA has played an important role in disease diagnosis and control, particularly in resource-constrained settings where resources are limited and point-of-care testing is critical to filling the gap.[17] Testing for the presence of an infectious agent in body fluids using LFA can be performed in two ways: lateral flow immunoassay (LFI) or lateral flow nucleic acid (LFNA). The immunoassay variant looks for antibodies created as a result of the presence of an infectious agent, whereas the nucleic acid variant is built to detect an amplified nucleic acid sequence specific to a target infectious agent.[18] Speaking specifically to the SARS-CoV-2 coronavirus, the antibody-based immunoassay method of lateral flow is predominantly used, targeting one of either a monoclonal antibody directed at a viral antigen, or a viral antigen that is recognizable by a patient's developed antibodies.[19][20]
Testing using LFI is generally as follows. A specialized adsorbent sample pad at one end of the LFI strip receives the specimen material. That material then migrates to the next conjugate release pad, where the specimen material is exposed to "antibodies that are specific to the target analyte and are conjugated to coloured or fluorescent particles."[13] The material then progresses to a detection zone containing antibodies or antigens that are fixed in the zone and intended to react to a specific analyte. If the analyte is present, a test line produces a visual, qualitative response, and a control line ensures proper liquid flow across the strip. A wicking pad at the other end properly maintains the flow of liquids across the strip.[13]
2.2.1 Severe acute respiratory syndrome (SARS)
Severe acute respiratory syndrome, otherwise known as SARS, arose in South China in late 2002. Caused by the SARS coronavirus (SARS-CoV) and believed to have originated from horseshoe bats[21], SARS eventually was contained in the summer of 2003. The last known infection was in April 2004, due to a laboratory accident.[22] During that time, the following sample collection and test procedures evolved from the related outbreaks (note that this is only a summary; consult the cited literature directly for full details)[23][24][25][26][27]:
- Determine that the patient is indicating clinical and/or epidemiological evidence of SARS (meets case definitions). As Knobler et al. put it: "SARS-CoV testing should be considered if no alternative diagnosis is identified 72 hours after initiation of the clinical evaluation and the patient is thought to be at high risk for SARS-CoV disease (e.g., is part of a cluster of unexplained pneumonia cases)."[25]
- Collect multiple specimen types at different time points of the patient's illness. Respiratory and plasma or serum specimens should be collected early into the first week of illness. Respiratory samples should be from nasopharyngeal aspirates and swabs of the upper respiratory tract or, in some cases, fluids from the lower respiratory tract using bronchoalveolar lavage, tracheal aspiration, or a pleural tap. (Sputum can also be collected.) Whole blood (5 to 10 ml) is collected into either a serum separator tube for blood serum or EDTA tube for blood plasma. Stool samples are also of import early on for virus isolation or detection and are useful in at least the first and second weeks of the illness. Blood serum is useful in weeks two and three for detecting a rising titre. Additionally, the literature also makes reference to methods of collecting specimens postmortem.
- Conduct testing. At the time, the two primary test types used were enzyme immunoassay (EIA; today more commonly known as ELISA[28]) for detection of serum antibody and reverse transcription polymerase chain reaction (RT-PCR) for detection of the virus' RNA. The U.S. Centers for Disease Control and Preventions had this to say about these tests in May 2004[24]:
Both the EIA and the RT-PCR tests are sensitive and highly specific for SARS-CoV. The ability to diagnose SARS-CoV infection in a patient is often limited, however, by either the low concentration of virus in most clinical specimens (RT-PCR assays) or the time it takes a person to mount a measurable antibody response to SARS-CoV (serologic assays). The likelihood of detecting infection is increased if multiple specimens (e.g., stool, serum, respiratory tract specimens) are collected at several times during the course of illness.
- The literature also makes reference to an immunofluorescence assay (IFA) for detecting antibody, with the Centers for Disease Control and Prevention (CDC) calling its results "essentially identical to those for the EIA for SARS antibody."[24] Tangentially, isolation of SARS-CoV in cell culture from a clinical specimen is also referenced, though such activity is reserved for Biosafety Level 3 (BSL-3) laboratories.
- Confirm the results. Laboratory confirmation is based on one of 1. initial local lab detection and subsequent national reference lab confirmation of a validated serology-based test detection; 2. isolation of SARS-CoV in cell culture with subsequent confirmation from a validated test; or 3. initial local lab detection and subsequent national reference lab confirmation of SARS-CoV RNA from a validated RT-PCR test which used either two clinical specimens from different sources or two same-source clinical specimens from two different days.
- Additionally, in the case of serology, one of the following must be true:
- SARS-CoV serum antibodies are detected in a single serum specimen; or,
- a "four-fold or greater increase in SARS-CoV antibody titer between acute- and convalescent-phase serum specimens tested in parallel"[24] is detected; or,
- a "negative SARS-CoV antibody test result on acute-phase serum and positive SARS-CoV antibody test result on convalescent-phase serum tested in parallel"[24] is detected.
- Of note is the World Health Organization's (WHO) January 2004 cautionary message about serological diagnostics in not only SARS-CoV but other types of coronaviruses. At that time, they showed a level of unsurety in regards to how coronaviruses elicited serological cross-reactions and generated antigenic recall. They also preached caution in interpreting serological results in non-epidemic periods and when no viral sequence data are available. Finally, they also mentioned the added difficulties of rate cases when coinfection with a related human coronavirus occurs, "although the use of expressed proteins in Western blots may help to sort this out."[26] More than 15 years later, Loeffelholz and Tang put this concept into clearer terms, indicating that while "serological assays are not routinely used for diagnosis of [human coronavirus] infections due to the lack of commercial reagents," they still have important value "for understanding the epidemiology of emerging [human cornaviruses], including the burden and role of asymptomatic infections," as well as for antibody detection of novel and emerging coronaviruses.[29]
- Arrange for confirmatory testing to be performed by an appropriate test site in the case of a positive RT-PCR test.
- Report to state or local health departments details of patients radiographically confirmed with pneumonia with at least one SARS-CoV risk factor for exposure, clusters of healthcare workers with unexplained pneumonia, and any positive SARS-CoV test results. Additional international reporting of SARS by WHO Member States in regards to probable and laboratory-confirmed cases is also requested.
- Send off for an additional verification by an external member of the WHO's SARS Reference and Verification Laboratory Network before internationally announcing results as a laboratory-confirmed case.
2.2.2 Middle East respiratory syndrome (MERS)
Unlike SARS, Middle East respiratory syndrome, or MERS, continues to appear in the human population. Since its appearance in 2012, several thousand laboratory-confirmed cases of MERS have been reported to the WHO.[30] The virus MERS-CoV is believed to have originated from bats, which at some unknown point spread to Dromedary camels. Approximately 55 percent of MERS-CoV infections have come from direct contact with such camels, though it's not entirely clear how the rest of known cases have been caused[31] (Alshukairi et al. suggest asymptomatic or mildly symptomatic camel workers may serve as a possible transmission source[32]). The following sample collection and test procedures have evolved from working with the MERS-CoV virus (note that this is only a summary; consult the cited literature directly for full details)[33][34][35][36][37]:
- Determine that the patient is indicating clinical and/or epidemiological evidence of MERS (meets case definitions). "Testing for other respiratory pathogens using routinely available laboratory procedures, as recommended in local management guidelines for community-acquired pneumonia, should also be performed but should not delay testing for MERS-CoV."[35]
- Collect at a minimum both lower respiratory and upper respiratory tract samples. Lower respiratory tract specimens are typically the most revealing, as they have been shown to contain the highest viral load (due to the expression of the virus's cellular receptor DPP4 in the lower respiratory system). Bronchoalveolar lavage, tracheal aspiration, or a pleural tap can be used to collect specimens from the lower respiratory tract. (Sputum can also be collected.) Upper respiratory tract specimens (in this case, both a nasopharyngeal and an oropharyngeal swab are recommended) are also valuable in diagnosis, though extra care should be taken to ensure nasopharyngeal swabs gather secretions from the nasopharynx and not just the nostril. Nasopharyngeal aspiration is also an acceptable sample collection method for the upper respiratory tract.
- Regarding serum specimens, slight differences in guidance appear between WHO guidance and CDC guidance. The WHO appears to differentiate between symptomatic and asymptomatic patient testing, whereas the U.S. CDC seems to only indirectly differentiate the two. The WHO suggests if testing symptomatic patients, stick with lower and upper respiratory tract specimens, which will be tested using nucleic acid amplification (molecular) testing (NAAT). Serological testing of serum specimens should be used for symptomatic patients "only if NAAT is not available."[35] If this is the case, the WHO recommends paired samples, one collected within the first week of illness and the second about three to four weeks later. For asymptomatic patients in high-contact outbreak scenarios, the WHO recommends all three sample types (with respiratory samples taken preferably within 14 days of last documented contact).
- The current CDC guidance differentiates between molecular testing for active infections and serology for previous infections. The CDC adds that "MERS-CoV serology tests are for surveillance or investigational purposes and not for diagnostic purposes."[33] Whether or not to collect a serum specimen in MERS diagnostics may depend on the assay used, however. For example, the CDC, in its Version 2.1 guidance, indicates that testing using the CDC MERS rRT-PCR assay requires collection of serum in addition to upper and lower respiratory tract specimens. For that specific assay, the CDC differentiates between patients who've had symptom onset less than 14 days prior and those who've had it 14 days or later: if prior, serology is for the rRT-PCR test, and if later, serology is for antibody testing. In either case, 200 µL of serum is required.
- Conduct testing. NAAT methods like real-time reverse-transcription polymerase chain reaction (rRT-PCR) assays have been the most common tool for diagnosing MERS-CoV infection due to their high sensitivity. According to late 2018 research by Kelly-Cirino et al., at least 11 commercial single assay and five commercial multiplex assay kits are available (see Table S1, a PDF file, from their highly relevant paper), perhaps more as of April 2020. Serological antibody detection is performed using ELISA, indirect immunofluorescence (IIF), and microneutralization.
- Confirm the results. Laboratory confirmation of MERS-CoV infection is the same for both the WHO and the CDC: one of either a validated NAAT test providing a positive result for at least two different genomic targets, or a validated NAAT test providing a positive result for a specific genomic target along with sequencing confirmation of a separate genomic target. Persons under investigation who receive one negative NAAT result on a recommended specimen are considered to be negative for active MERS-CoV infection. The laboratory should consider testing additional specimens after the first negative. The CDC considers known MERS patients to be negative for active MERS-CoV infection after two consecutive negative NAAT tests on all specimens. The WHO adds: "A patient with a positive NAAT result for a single specific target without further testing but with a history of potential exposure and consistent clinical signs is considered a probable case."[35] The WHO also has additional guidance on using serology for confirming MERS-CoV infection for purposes of reporting under the International Health Regulations.
- Report using national reporting requirements. More broadly, state or local health departments should receive details about received specimens to be tested for MERS-CoV, even before testing begins. Regardless of result, the final positive or negative laboratory confirmation should also be reported to national authorities. If the infection becomes widespread, updates for each new confirmed case or suspected positive should also be made.
2.2.3 The common cold
Approximately 10 to 15 percent of cases of what we call the "common cold" are associated with an endemic coronavirus, of which are two distinct groups: HCoV-229E and HCoV-OC43.[38] Disease symptoms associated with these coronaviruses—typically in the form of respiratory infection and the symptoms that come with it—by themselves are typically mild[29], and laboratory testing isn't necessarily indicated for those immunocompetent individuals capable of self-limiting.[39] However, symptom overlap with pharyngitis and bronchitis, as well as the complication of pharyngitis and sinusitis also potentially having bacterial origin, can complicate clinical diagnosis. Additionally, as more antivirals that target a specific virus are created, and as concerns of unnecessarily using antibiotics to treat viral diseases grows[40][41], laboratory methods of respiratory virus diagnosis—particularly for those who are immunocompromised—have value.[38][39]
RT-PCR, a molecular method, has been used for well over a decade for detecting coronaviruses.[38][42] However, as molecular methods of analysis have expanded over the years, more rapid solutions for testing have been developed. For example, the GenMark ePlex rapid multiplex molecular diagnostics instrument and the ePlex Respiratory Pathogen Panel were evaluated in a multicenter trial by Babady et al. in 2017.[39] The panel is capable of testing for the presence of 15 viral types—including the -229E, -OC43, and two other coronaviruses—and two bacterial types in nasopharyngeal swab specimens, with results in typically less than two hours.[39] The costs associated with these sorts of tests, compared to their benefits, likely limits ubiquitous use at the first sign of a cold[39], but as molecular diagnostic technologies become more compact and easy-to-use, testing for infection by endemic human coronaviruses may become slightly more commonplace. However, as the authors point out, with no treatment for these endemic coronaviruses, any additional utility beyond diagnosing an illness as viral rather than bacterial would primarily be found in epidemiological studies of the associated genotyping data.[39]
2.3 Organizational and agency guidance on COVID-19 testing
NOTE: Information shown here may rapidly become outdated given how quickly response to pandemic testing can change. A full attempt to keep the content relevant will be made.
Laboratory guidance for testing for SARS-CoV-2 has been relatively quick to evolve. The timely development and organized use of accurate assays and meaningful screening protocols, however, has been inconsistent worldwide, with some countries more urgently and agilely responding than others.[43][44][45] With any novel virus, clinicians and public health experts are dealing with unknown factors. However, public health organizations and agencies have had a base to work from when creating laboratory testing guidance for a novel coronavirus, with more than 40 years of experience with coronavirus biology, pathogenesis, and diagnosis.[46] And while there are fundamental differences between SARS-CoV-2 and its predecessor SARS-CoV, they still share approximately 70 to 80 percent of their genetic code.[47][48] In fact, the WHO had draft guidance for laboratory testing out as early as January 10, 2020, before gene sequencing was even completed.[49] This guidance and similar draft guidance from national public health organizations and agencies have received steady revisions since as understanding of the virus has grown.
Similar to its predecessors SARS-CoV and MERS-CoV, RT-PCR is being recommended in guidance for detecting SARS-CoV-2's RNA in specimens and thus laboratory confirmation of COVID-19 cases. Serology has its place in testing as well, though with similar lessons from SARS and MERS that it's best used to test for past infection (typically after 14 days of suspected contact with a carrier, or mild symptoms) and thus potential short-term immunity due to the presence of antibodies in blood. In its March 19 guidance, the WHO said: "In cases where NAAT assays are negative and there is a strong epidemiological link to COVID-19 infection, paired serum samples (in the acute and convalescent phase) could support diagnosis once validated serology tests are available."[50] On April 3, the U.S. Food and Drug Administration approved the countries first COVID-19 serology test created by Cellex, though Mayo Clinic was also on the verge of rolling out its own in-house serology test as well[51] As of April 28, the U.S. FDA has granted emergency use authorizations (EUA) for eight serology tests.[52] (Note: Johns Hopkins appears to be maintaining a page tracking approved serology tests around the world.)
The following sample collection and test procedures have evolved from the COVID-19 pandemic (note that this is only a summary; consult the cited literature directly for full details)[50][53][54][55][56]:
- Determine that the patient is indicating clinical and/or epidemiological evidence of COVID-19 (meets case definitions). Case definitions and testing criteria have initially been strict, but as the CDC notes, as test kit availability ramps up, it "will allow clinicians to consider COVID-19 testing for a wider group of symptomatic patients."[53] However, clinicians are still encouraged to consider other causes for respiratory illness. The CDC provides a priority list, making hospitalized patients and symptomatic healthcare workers the top priority, followed by "those who are at highest risk of complication of infection," then "individuals in the surrounding community of rapidly increasing hospital cases." In the future, as more serology options and other resources become available, serological surveys of people who have never been diagnosed (asymptomatic or otherwise) may begin.[57][58]
- Collect at a minimum upper respiratory tract specimens and, whenever possible, lower respiratory tract specimens. Although too early to say with certainty, it appears lower respiratory tract specimens such as sputum and bronchoalveolar lavage fluid are typically the most reliable specimen type for RT-PCR applications, as they have been shown to contain the highest viral load, in comparison to upper respiratory tract specimens.[59][60] As Wang et al. point out, "testing of specimens from multiple sites may improve the sensitivity and reduce false-negative test results,"[59] which is largely reflected in WHO, CDC, Public Health England (PHE), and Public Health Laboratory Network (PHLN; Australia) testing guidance.
- Slight differences in upper respiratory tract specimen collection procedures can be found between the WHO/CDC and PHE/PHLN. Both the WHO and CDC offer nasopharyngeal and oropharyngeal swabs as options. The WHO doesn't appear to give a preference, whereas the CDC has a preference for nasopharyngeal swabs but maintains oropharyngeal as still remaining "an acceptable specimen type."[54] In comparison, the latest PHE and PHLN guidance prefer the approach of collecting from both pharynx locations—even with the same swab—"to optimize the chances of virus detection."[56] Nasopharyngeal aspiration is also an acceptable sample collection method for the upper respiratory tract according to all mentioned entities, though the PHLN specifies that it is a substitution for only the nasopharyngeal (they now refer to it as "deep nasal") specimen.[56]
- Regarding serum specimens, statements differ slightly. The WHO notes serology to be useful for retrospective case definition, using paired specimens from the acute and convalescent phases of the disease. The CDC doesn't make reference to serum or serology in their clinical specimen guidance. The PHE suggests hospital patients have "a sample for acute serology" taken but say little else.[55] The PHLN gives similar advice as the WHO, while emphasizing a need "to facilitate retrospective testing, if this is relevant, once serology tests become available."[56]
- Finally, and more recently, potential evidence of saliva having diagnostic value for detecting SARS-CoV-2 has arisen. As Xu et al. note in their mid-April paper, the "diagnostic value of saliva specimens for ... nucleic acid examination remains limited but promising."[61] Another paper awaiting peer review by researchers at Yale School of Public Health provides similiar thoughts, though is generally more optimistic than the paper published by Xu et al., suggesting salive from the opening of the mouth (in contrast to Xu et al. and their finding of better results from salive in the throat) may be viable specimen.[62] In fact, an April EUA by the FDA has been made for the first saliva-based COVID-19 test, produced by Vault Health, Inc.[63] As these and similar studies get peer reviewed and methods validated, preferred sample types (and the guidance recommending them) may change. Stay aware of the evolving science.
- Conduct testing. NAAT methods like rRT-PCR have been the primary tools for diagnosing SARS-CoV-2 infection due to their high sensitivity. The PHLN provides the most background about PCR in their guidance, noting that laboratories in its network are confirming positive infections "either with RT-PCR assays detecting a different target gene, or broadly reactive PCR tests with sequencing of amplicons."[56] The latter option is less common due to long turnaround time. They also note that other zoonotic viruses such as SARS-CoV are capable of being detected from PCR assays, though endemic coronaviruses like -229E won't be. The WHO, CDC, and PHLN underscore the idea that viral cultures for routine diagnoses are not practical and, if attempted, should only be performed in Biosafety Level 3 (BSL-3) laboratories. As of April 5, no specific methods have been suggested for serological antibody detection, though the current set of approved serology tests from around the world appear to use lateral flow immunoassay, ELISA, or neutralization methods.[58]
- Confirm the results. The strongest public guidance for considering a potential case as being laboratory-confirmed for SARS-CoV-2 infection comes from the WHO. In their guidance, they differentiate between cases by NAAT "in areas with no known COVID-19 virus circulation" and "in areas with established COVID-19 virus circulation." In the first case, one of these conditions must apply: either a validated NAAT test providing a positive result for at least two different genomic targets, or a validated NAAT test providing a positive result for the presences of betacoronavirus along with sequencing confirmation of a separate genomic target, "as long as the sequence target is larger or different from the amplicon probed in the NAAT assay used."[50] In the latter case of established virus circulation, the WHO notes that "a simpler algorithm might be adopted in which, for example, screening by rRT-PCR of a single discriminatory target is considered sufficient."[50] However, if testing produces one or more negative results, that doesn't necessarily rule out SARS-CoV-2 infection. If suspicion of infection remains high, particularly if only upper respiratory tract specimens were collected, additional specimens from the lower respiratory tract should be collected and analyzed. They also emphasize that both external and internal controls should be applied to NAAT runs.
- Report using state and, if applicable, national reporting requirements. (See the next chapter for more on reporting.) Regardless of result, the final positive or negative laboratory confirmation should also be reported to state and national authorities. In the U.S., for example, this means reporting to the local or state health department using the CDC's PUI and Case Report Form. In Canada, reports are sent to the Public Health Agency of Canada (PHAC) via their Coronavirus Diseases (COVID-19) Case Report Form.
2.4 Current test methods and their differences
NOTE: Information shown here may rapidly become outdated given how quickly response to pandemic testing can change. A full attempt to keep the content relevant will be made.
2.4.1 Background on the laboratory testing environment
Before continuing, it should be noted that many elements of the prior-mentioned COVID-19 testing guidance have governmental public health laboratories in mind. However, as the scale of the epidemic has grown, the need for commercial laboratories and assay developers to get involved with increasing analytical testing throughput—through a more rigorous public-private partnership—has become abundantly clear.[64][65][66][67] Even so, at least in the United States, turnaround times have been slow due to a variety of factors, from lack of in-house laboratory resources to handle high test volumes and a slower-than-expected ramping up of test kit production[64][66][67], to actually getting diagnostic assays that are more rapid (yet still accurate) in their diagnosis, simpler to use, and useable at the point of care.[68][69] The good news is examples of these rapid point-of-care molecular test kits are now becoming available around the globe, including the United States as part of the U.S. Food and Drug Administration's EUA process.
As the demand for expanded diagnostic testing grows in the face of a pandemic, it's important to compare the U.S. laboratory testing environments of public health and large commercial testing labs with those of small, in-office clinical labs. In the U.S., all but research-based laboratory testing of human specimens is regulated under the Clinical Laboratory Improvement Amendments (CLIA), including public health laboratories. CLIA is meant to ensure quality laboratory testing in the country. Seven different criteria are used to gauge and assign one of three complexity levels to laboratory devices and assays: high, moderate, and waived.[70][71] Additionally, CLIA mandates clinical laboratories handling specimens originating from the U.S. and its territories to apply for a CLIA certificate that is appropriate for the type of testing it performs. Labs using complex devices and assays would have to apply for a high complexity certificate, and so on. Waived tests are recognized as simple to perform with a low risk of erroneous results and include among others urinalysis for pregnancy and drugs of abuse, blood glucose and cholesterol tests, and fertility analysis.
Of the more than 256,000 non-exempt CLIA-registered labs in the U.S., only 33,212 or 13 percent of them are certified to perform moderate- and high-complexity testing.[72] Your public health labs and commercial diagnostic labs fall into this category, with investments in the personnel, training, certifications, and equipment to conduct those sorts of tests. Contrast this with the small yet numerous physician office laboratories (POLs) and how they operate. As of October 2019, nearly 46 percent of CLIA-certified laboratories in the United States are POLs.[72] Located in an ambulatory or outpatient care setting, these labs test specimens from human patients to assist with the diagnosis, treatment, or monitoring of a patient condition. Testing in the clinical lab generally depends on three common methodologies to meet those goals: comparing the current value of a tested substance to a reference value, examining a specimen with microscopy, and detecting the presence of infection-causing pathogens.[73]
These POL's operate in a different environment than your average public health laboratory or reference lab that receives, processes, and reports on specimens en masse. The POL is typically a smaller operation, performing simple laboratory testing that can produce useful diagnostic data cheaply and rapidly. Rather than performing advanced pathology and molecular diagnostic procedures that require specific equipment and expertise, the POL typically focuses on blood chemistry, urinalysis, and other testing domains that don't require significant resources and provide rapid results. This can be seen in Centers for Medicare and Medicaid Services statistics reported in October 2019 that show nearly 67 percent of POLs in the U.S. are certified to provide CLIA-waived tests[72], "simple tests with a low risk for an incorrect result."[70]
As of the end of April, with 1. all but a handful of the current EUAed molecular in vitro diagnostic COVID-19 test kits being limited to moderate- and high-complexity CLIA labs[52] (the FDA claims that EUAed SARS-CoV-2 tests authorized for "use at the point of care" are considered CLIA-waived tests[74]), and 2. local government entities such as New York City's Department of Health and Mental Hygiene sending reminders to local laboratories that serology testing (as of April 7) is also still considered high-complexity in nature[75] (though the FDA has EUAs for serology tests that are approved for both moderate and complex CLIA labs[52]), a significant majority of clinical laboratories are shut out from assisting with the effort to test the U.S. population for SARS-CoV-2 infection. Given the rapid rate of change at multiple levels of government and society, and wildly varying levels of reliable information being given to the public[76][77], it's important to remember these fundamental differences in laboratories when trying to explain to someone why they are, as of yet, unable to go to their primary care physician and get tested for SARS-CoV-2 in the doctor's office. Should researchers develop and the FDA provide EUAs for more CLIA-waived point-of-care assays, these differences may become less noticeable, and more people will be able to be tested.
2.4.2 PCR-based
As of April 28, the U.S. Food and Drug Administration (FDA) has 42 EAUs for molecular in vitro diagnostic test kits. Forty-one of those 42 test kits use some form of RT-PCR methods, with most using real-time versions of RT-PCR (rRT-PCR). Thirty of those 41 are only authorized to be used in CLIA-certified high-complexity laboratories, with nine being rated for both moderate- and high-complexity. Two RT-PCR kits—Mesa Biotech's Accula SARS-Cov-2 Test and Cepheid's Xpert Xpress SARS-CoV-2 Test—have an additional authorization for point-of-care (POC) use (and thus CLIA-waived use) when used with their authorized POC devices.[52]
Of course, there are many more test kits than those approved in the United States. The Foundation for Innovative New Diagnostics (FIND) is currently "collating an overview of all SARS-CoV-2 tests commercially available or in development for the diagnosis of COVID-19."[78] As of April 28, their site shows more than 180 commercialized manual NAAT tests around the world (most being RT-PCR), with more than 20 in development.
2.4.3 LFA- and LAMP-based
LFAs are currently rare, but due to their advantages of being quick and useable at the point of care, some have suggested that as a format for antigen and antibody (serology) testing, they could positively change the testing landscape.[19][79][80] As of April 28, only three LFA serology tests have received EUAs by the FDA for moderate- and high-complexity CLIA-certified labs. An article by Sheridan in Nature Biotechnology highlights a handful of others developed around the world (see their Table 1).[19] FIND shows more than 120 commercialized rapid diagnostic immunoassay tests around the world, though it's not clear how many of them actually LFAs (from their list, only one is explicitly stated as being LFA).[78] At this point, it's safe to say that LFA are still being developed, and it may be May or June before we start seeing more of them, at least in the United States.[79]
Also of note is the LAMP (and RT-LAMP) method. Abbott's ID NOW COVID-19 test is described by the FDA as using "isothermal nucleic acid amplification technology for the qualitative detection of SARS-CoV-2 viral nucleic acids."[81] This is presumably a loop-mediated isothermal amplification or LAMP test, the only one so far approved by the FDA. Among FIND's list of more than 180 commercialized manual NAAT tests around the world, five of them are explicitly shown to be some form of LAMP test. Multiple preprint papers on medRxiv and bioRxiv suggest that RT-LAMP could provide rapid results for SARS-CoV-2 testing[82][83][84][85], and Abbott is stating its EUAed ID NOW COVID-19 test can be completed within five minutes[86], providing further optimism for rapid point-of-care testing in the near future.
2.4.4 Blood serum
Blood serum or serology assays come in three common varieties: LFA, enzyme-linked immunosorbent assay (ELISA), or neutralization assay.[58] As discussed prior, LFAs are intended to be rapid point-of-care tools for qualitatively testing body fluids for patient antibodies or viral antigen. The ELISA is, in contrast, a more lab-bound method which produces results that are qualitative or quantitative. In the context of COVID-19 testing, ELISA tests for the presence of patient antibodies in a given specimen based upon whether or not an interaction is observed with the viral proteins present on the test plate. However, even if antibodies are present, ELISA isn't able to tell a clinician if those antibodies are able to protect against future infection. Neutralization assays are the lengthiest to complete, taking from three upwards to five days.[58] This is largely due to the fact that the assay depends on culturing cells that encourage growth of the target virus. Afterwards, introduced patient antibodies, if present, will fight to prevent viral infection of cells. This process is performed in decreasing concentrations, giving the clinician an opportunity to "visualize and quantify how many antibodies in the patient serum are able to block virus replication."[58] In contrast to ELISA, a neutralization assay is able to determine if a patient's antibodies are actively fighting against the target virus, even after recovering from the infection.
Johns Hopkins' Center for Health Security appears to be tracking serology-based COVID-19 tests that are in development or have been approved in various parts of the globe. However, for the most up-to-date list of serology tests that have received EUAs in the United States, the FDA's EUA list appears to be the best source. As of April 28, the FDA shows eight serology assays approved for diagnostic use in the U.S. Of those eight, three appear to be LFAs and the other five ELISA-based.
A review of Johns Hopkins' tracking list in early April showed more LFA-based tests among those approved in other parts of the world. ScanWell Health's kit appears to be proprietary, but given its claims of rapid results, it's probably LFA. A Singapore-based operation has two tests, one that is advertised as rapid and another as a neutralization test. Of the 22 assays approved for research or surveillance, six appear to be based on ELISA, one is unknown, and the rest are LFA-based assays. Among those still in development, an LFA stands out for integrating CRISPR detection.[87] CRISPR (clustered regularly interspaced short palindromic repeats) represents bacterial and archaeal DNA sequences derived from DNA fragments of previous infection. This genetic material can then be used as an activator of biomarkers when attached RNA "guides" find a match with target viral RNA in patient specimen.[88]
2.4.5 Testing alternatives and challenges
We’ve never faced this before, where clinical labs needed to very quickly be able to ramp up a test so fast.[89]
- Jennifer Doudna, Executive Director of the Innovative Genomics Institute, University of California, Berkeley
Though the dismantling and fund-cutting (proposed and real) of government programs designed to protect the populace from pandemics—as well as shortfalls in funding overall[90]—have likely hobbled local, national, and global response to COVID-19[91][92][93][94], it should be recognized that this pandemic may arguably represent a once-in-a-century type of event.[95][96] That said, even the most well-prepared governments would still face challenges in quickly learning about, controlling, and developing therapies for a novel disease agent. Shortages in supplies, workers, funding, and other resources are inevitably caused with a pandemic as people across all types of infrastructure fall ill.[90][97] This requires the additional human elements of adaptability, drive, and shared knowledge to find new and alternative solutions to fighting the challenges inherent to fighting against a novel disease.
See for example a non-peer-reviewed paper published on bioRxiv in early April 2020, where Schmid-Burgk et al. point out that though RT-PCR methods are the most common for currently testing for SARS-CoV-2, "global capacity for testing using these approaches, however, has been limited by a combination of access and supply issues for reagents and instruments." They propose "a novel protocol that would allow for population-scale testing using massively parallel RT-LAMP by employing sample-specific barcodes." They claim that a single heating step, pooled processing, and parallel sequencing with computational analysis would allow for the testing and tacking of "tens of millions of samples." Though the protocol has not been validated with clinical samples, and concerns about sensitivity levels of RT-LAMP (an isothermal nucleic acid amplification technique that allows for RNA amplification) have been raised, the authors' work exemplifies the immediacy and ingenuity going into finding workable solutions to a once-a-century problem.[82]
Another example of ingenuity in the face of difficult circumstances can be found at the University of California, Berkeley. Its Innovative Genomics Institute (IGI) has rapidly repurposed a 2,500-square-foot scientific lab into an automated diagnostic laboratory that can initially process more than 1,000 patient samples per day, with the ability to ramp up to 3,000 per day thanks to robotics and a streamlined workflow. Partnering with dozens of people from Thermo Fisher Scientific, Salesforce, Third Wave Analytics, and Hamilton Corp., the lab is focused on not only turnaround time but also accuracy of results through automation. Their continued success, of course, relies on a steady supply of reagents and related supplies from Thermo Fisher.[89][98]
Others have also expressed concerned about the global supply of reagents necessary to test for SARS-CoV-2. Successful testing using RT-PCR requires two different enzymes: reverse transcriptase, for converting RNA to DNA, and polymerase, for amplifying the converted DNA. These enzymes and other reagent components may be instrument-specific, and at least one component has to be sympathetic to detection of the target virus' RNA. Little of this can be prepared without a proper sequence of the virus in question. Dr. Ronald Leonard, president and medical director of Cytocheck Laboratory and medical director of the Labette Health hospital, has expressed the difficulties associated with reagent manufacturing thusly[99]:
With the instant demand for SARS-CoV-2 testing, the manufacturing process had to start from scratch for the SARS-CoV-2 specific components, and this did cause a lag time before reagents were available. The increased demand coupled with the decision to only allocate reagents to two national laboratories, some state health departments, and to "hot spots" has compounded the difficulty for laboratories like ours to obtain the necessary reagents to perform the testing.
Reports of reagent shortages appeared in March and April from various sources[100][101][102][103], though whether the shortage is a real supply issue or "a consequence of restrictive policies on where and how testing could be completed" is argued by some.[99][102] Others have taken matters into their own hands in regards to reagent component shortages. Noting Irish laboratories' difficulties sourcing lysis buffer (for isolating molecules of interest and keeping them stable), Cork Institute of Technology's Dr. Brigid Lucey worked with several other virologists and microbiologists, as well as pharmaceutical company Eli Lilly, to produce a custom-formulated yet high-quality lysis buffer for not only Irish laboratories but also other countries can take advantage of. "We are happy to share what we found with other countries and it’s important our scientists retain their skills to make this kind of formulation because we may need to do this again in the future if we get other pandemics," she said.[103]
References
- ↑ AdvaMedDx (2013). "Introduction to Molecular Diagnostics: The Essentials of Diagnostics Series". pp. 19. https://dx.advamed.org/research/resource-center/introduction-molecular-diagnostics-essentials-diagnostics-series-pdf. Retrieved 08 April 2020.
- ↑ Gregg, A.R. (2018). "Expanded Carrier Screening". Obstetrics and Gynecology Clinics of North America 45 (1): 103–112. doi:10.1016/j.ogc.2017.10.005. PMID 29428278.
- ↑ Chokoshvili, D.; Vears, D.F.; Borry, P. (2018). "Reproductive autonomy in expanded carrier screening: More than meets the eye?". Expert Review of Molecular Diagnostics 18 (12): 993–94. doi:10.1080/14737159.2018.1544496. PMID 30394810.
- ↑ Edgerly, C.H.; Weimer, E.T. (2018). "The Past, Present, and Future of HLA Typing in Transplantation". Methods in Molecular Biology 1802: 1–10. doi:10.1007/978-1-4939-8546-3_1. PMID 29858798.
- ↑ Loda, M. (1994). "Polymerase chain reaction-based methods for the detection of mutations in oncogenes and tumor suppressor genes". Human Pathology 25 (6): 564–71. doi:10.1016/0046-8177(94)90220-8. PMID 7912220.
- ↑ Ninfa, A.J.; Ballou, D.P.; Benore, M. (2009). Fundamental Laboratory Approaches for Biochemistry and Biotechnology. Wiley. pp. 408–410. ISBN 9780470087664. https://books.google.com/books?id=k6_XQwAACAAJ&pg=PA408. Retrieved 08 April 2020.
- ↑ Hamborsky, J.; Kroger, A.; Wolfe, C., ed. (2015). Epidemiology and Prevention of Vaccine-Preventable Diseases (13th ed.). Centers for Disease Control and Prevention. https://www.cdc.gov/vaccines/pubs/pinkbook/index.html. Retrieved 08 April 2020.
- ↑ Buckingham, L. (2019). "Chapter 11: Detection and Identification of Microorganisms". Molecular Diagnostics: Fundamentals, Methods and Clinical Applications (3rd ed.). F.A. Davis Company. pp. 301–343. ISBN 9780803699540. https://books.google.com/books?hl=en&lr=&id=dJWNDwAAQBAJ&pg=301.
- ↑ Kim, H.; Kang, N.; An, K. et al. (2017). "MRPrimerV: A database of PCR primers for RNA virus detection". Nucleic Acids Research 45 (D1): D475–81. doi:10.1093/nar/gkw1095. PMC PMC5210568. PMID 27899620. https://www.ncbi.nlm.nih.gov/pmc/articles/PMC5210568.
- ↑ Makin, S. (5 February 2020). "How Coronaviruses Cause Infection—from Colds to Deadly Pneumonia". Scientific American. https://www.scientificamerican.com/article/how-coronaviruses-cause-infection-from-colds-to-deadly-pneumonia1/. Retrieved 08 April 2020.
- ↑ Rohde, R. (31 January 2020). "2019 Novel Coronavirus (2019-nCoV) Update: Uncoating the Virus". American Society for Microbiology. https://asm.org/Articles/2020/January/2019-Novel-Coronavirus-2019-nCoV-Update-Uncoating. Retrieved 08 April 2020.
- ↑ Hadaya, J.; Schumm, M.; Livingston, E.H. (2020). "Testing Individuals for Coronavirus Disease 2019 (COVID-19)". JAMA. doi:10.1001/jama.2020.5388. PMID 32236503.
- ↑ 13.0 13.1 13.2 13.3 13.4 Koczula, K.M.; Gallotta, A. (2016). "Lateral flow assays". Essays in Biochemistry 60 (1): 111–20. doi:10.1042/EBC20150012. PMC PMC4986465. PMID 27365041. https://www.ncbi.nlm.nih.gov/pmc/articles/PMC4986465.
- ↑ Liu, Z.; Hu, J.; Qu, Z.; Xu, F. (2018). "Chapter 8: Paper-Based Immunoassays". In Vashist, S.K.; Luong, J.H.T.. Handbook of Immunoassay Technologies: Approaches, Performances, and Applications. Academic Press. pp. 183–202. ISBN 9780128117620. https://books.google.com/books?id=jSk0DwAAQBAJ&pg=PA183. Retrieved 08 April 2020.
- ↑ Jauset-Rubio, M.; Svobodová, M.; Mairal, T. et al. (2016). "Ultrasensitive, rapid and inexpensive detection of DNA using paper based lateral flow assay". Scientific Reports 6: 37732. doi:10.1038/srep37732. PMC PMC5123575. PMID 27886248. https://www.ncbi.nlm.nih.gov/pmc/articles/PMC5123575.
- ↑ Foysal, K.H.; Seo, S.E.; Kim, M.J. et al. (2019). "Analyte Quantity Detection from Lateral Flow Assay Using a Smartphone". Sensors 19 (21): 4812. doi:10.3390/s19214812. PMC PMC6864604. PMID 31694281. https://www.ncbi.nlm.nih.gov/pmc/articles/PMC6864604.
- ↑ Hanafiah, K.M.; Arifin, N.; Bustami, T. et al. (2017). "Development of Multiplexed Infectious Disease Lateral Flow Assays: Challenges and Opportunities". Diagnostics 7 (3): 51. doi:10.3390/diagnostics7030051. PMC PMC5617951. PMID 28880218. https://www.ncbi.nlm.nih.gov/pmc/articles/PMC5617951.
- ↑ Ngom, B.; Guo, Y.; Wang, X.; Bi, D. (2010). "Development and application of lateral flow test strip technology for detection of infectious agents and chemical contaminants: A review". Analytical and Bioanalytical Chemistry 397: 1113–1135. doi:10.1007/s00216-010-3661-4. PMID 20422164.
- ↑ 19.0 19.1 19.2 Sheridan, C. (23 March 2020). "Fast, portable tests come online to curb coronavirus pandemic". Nature Biotechnology - News. doi:10.1038/d41587-020-00010-2. https://www.nature.com/articles/d41587-020-00010-2. Retrieved 08 April 2020.
- ↑ McKie, R. (9 December 2017). "Scientists trace 2002 Sars virus to colony of cave-dwelling bats in China". The Guardian. https://www.theguardian.com/world/2017/dec/10/sars-virus-bats-china-severe-acute-respiratory-syndrome. Retrieved 03 April 2020.
- ↑ Normile, D. (2004). "Mounting Lab Accidents Raise SARS Fears". Science (5671): 659–61. doi:10.1126/science.304.5671.659. PMID 15118129.
- ↑ New York State Department of Health (February 2004). "Laboratory Testing for SARS". State of New York. https://www.health.ny.gov/diseases/communicable/sars/sars_reporting/attachment_6_dear_doctor_lab.htm. Retrieved 03 April 2020.
- ↑ 24.0 24.1 24.2 24.3 24.4 Centers for Disease Control and Prevention (21 May 2004). "Public Health Guidance for Community-Level Preparedness and Response to Severe Acute Respiratory Syndrome (SARS), Version 2 - Supplement F: Laboratory Guidance" (PDF). Centers for Disease Control and Prevention. https://www.cdc.gov/sars/guidance/f-lab/downloads/F-lab-full.pdf. Retrieved 03 April 2020.
- ↑ 25.0 25.1 Knobler, S.; Mahmoud, A.; Lemon, S. et al., ed. (2004). "Appendix C: In the absence of SARS-CoV transmission worldwide: Guidance for surveillance, clinical and laboratory evaluation, and reporting". Learning from SARS: Preparing for the Next Disease Outbreak. National Academies Press. pp. 292–302. doi:10.17226/10915. ISBN 9780309182157.
- ↑ 26.0 26.1 World Health Organization (23 January 2004). "WHO SARS International Reference and Verification Laboratory Network: Policy and Procedures in the Inter-Epidemic Period". World Health Organization. http://www.who.int/csr/resources/publications/en/SARSReferenceLab.pdf. Retrieved 03 April 2020.
- ↑ Liang, G.; Chen, Q.; Xu, J. et al. (2004). "Laboratory Diagnosis of Four Recent Sporadic Cases of Community-acquired SARS, Guangdong Province, China". Emerging Infectious Diseases 10 (10): 1774–81. doi:10.3201/eid1010.040445. PMC PMC3323270. PMID 15504263. https://www.ncbi.nlm.nih.gov/pmc/articles/PMC3323270.
- ↑ Lequin, R.M. (2005). "Enzyme Immunoassay (EIA)/Enzyme-Linked Immunosorbent Assay (ELISA)". Clinical Chemistry 51 (12): 2415–18. doi:10.1373/clinchem.2005.051532. PMID 16179424.
- ↑ 29.0 29.1 Loeffelholz, M.J.; Tang, T.-W. (2020). "Laboratory diagnosis of emerging human coronavirus infections – The state of the art". Emerging Microbes & Infections 9 (1): 747–56. doi:10.1080/22221751.2020.1745095. PMID 32196430.
- ↑ Bernard-Stoecklin, S.; Nikolay, B.; Assiri, A. et al. (2019). "Comparative Analysis of Eleven Healthcare-Associated Outbreaks of Middle East Respiratory Syndrome Coronavirus (Mers-Cov) from 2015 to 2017". Scientific Reports 9: 7385. doi:10.1038/s41598-019-43586-9. PMC PMC6517387. PMID 31089148. https://www.ncbi.nlm.nih.gov/pmc/articles/PMC6517387.
- ↑ Banerjee, A.; Kulcsar, K.; Misra, V. et al. (2019). "Bats and Coronaviruses". Viruses 11 (1): E41. doi:10.3390/v11010041. PMC PMC6356540. PMID 30634396. https://www.ncbi.nlm.nih.gov/pmc/articles/PMC6356540.
- ↑ Alshukairi, A.N.; Zheng, J.; Zhao, J. et al. (2018). "High Prevalence of MERS-CoV Infection in Camel Workers in Saudi Arabia". mBio 9 (5): e01985-18. doi:10.1128/mBio.01985-18. PMC PMC6212820. PMID 30377284. https://www.ncbi.nlm.nih.gov/pmc/articles/PMC6212820.
- ↑ 33.0 33.1 Centers for Disease Control and Prevention (2 August 2019). "CDC Laboratory Testing for Middle East Respiratory Syndrome Coronavirus (MERS-CoV)". Centers for Disease Control and Prevention. https://www.cdc.gov/coronavirus/mers/lab/lab-testing.html. Retrieved 04 April 2020.
- ↑ Centers for Disease Control and Prevention (2 August 2019). "Interim Guidelines for Collecting, Handling, and Testing Clinical Specimens from Persons Under Investigation (PUIs) for Middle East Respiratory Syndrome Coronavirus (MERS-CoV) – Version 2.1". Centers for Disease Control and Prevention. https://www.cdc.gov/coronavirus/mers/guidelines-clinical-specimens.html. Retrieved 04 April 2020.
- ↑ 35.0 35.1 35.2 35.3 World Health Organization (January 2018). "Laboratory testing for Middle East Respiratory Syndrome Coronavirus: Interim guidance". WHO/MERS/LAB/15.1/Rev1/2018. World Health Organization. https://www.who.int/csr/disease/coronavirus_infections/mers-laboratory-testing/en/. Retrieved 04 April 2020.
- ↑ Al-Abdely, H.M.; Midgley, C.M.; Alkhamis, A.M. et al. (2019). "Middle East Respiratory Syndrome Coronavirus Infection Dynamics and Antibody Responses among Clinically Diverse Patients, Saudi Arabia". Emerging Infectious Diseases 25 (4): 753-766. doi:10.3201/eid2504.181595.
- ↑ Kelly-Cirino, C.; Mazzola, L.T.; Chua, A. et al. (2019). "An updated roadmap for MERS-CoV research and product development: focus on diagnostics". BMJ Global Health 4 (Suppl. 2): e001105. doi:10.1136/bmjgh-2018-001105. PMC PMC6361340. PMID 30815285. https://www.ncbi.nlm.nih.gov/pmc/articles/PMC6361340.
- ↑ 38.0 38.1 38.2 Wat, D. (2004). "The common cold: A review of the literature". European Journal of Internal Medicine 15 (2): 79–88. doi:10.1016/j.ejim.2004.01.006. PMID 15172021.
- ↑ 39.0 39.1 39.2 39.3 39.4 39.5 Babady, N.E.; England, M.R.; Jurcic Smith, K.L. et al. (2018). "Multicenter Evaluation of the ePlex Respiratory Pathogen Panel for the Detection of Viral and Bacterial Respiratory Tract Pathogens in Nasopharyngeal Swabs". Journal of Clinical Microbiology 56 (2): e01658-17. doi:10.1128/JCM.01658-17. PMC PMC5786739. PMID 29212701. https://www.ncbi.nlm.nih.gov/pmc/articles/PMC5786739.
- ↑ Jenison, R. (30 November 2016). "Rapid lab tests can help reduce antibiotic resistance". STAT. https://www.statnews.com/2016/11/30/antibiotic-resistance-molecular-diagnostics/. Retrieved 03 April 2020.
- ↑ Roy, K. (26 September 2018). "Rapid test for viral infections reduces unnecessary antibiotic prescribing". Healio. https://www.healio.com/infectious-disease/antimicrobials/news/online/%7B226c31f3-1d8e-4ffe-82b1-654cb37303c4%7D/rapid-test-for-viral-infections-reduces-unnecessary-antibiotic-prescribing. Retrieved 03 April 2020.
- ↑ Mahoney, J.B. (2008). "Detection of Respiratory Viruses by Molecular Methods". Clinical Microbiology Reviews 21 (4): 716–47. doi:10.1128/CMR.00037-07. PMC PMC2570148. PMID 18854489. https://www.ncbi.nlm.nih.gov/pmc/articles/PMC2570148.
- ↑ Subbaraman, N.; Callaway, E. (23 March 2020). "Coronavirus tests: Researchers chase new diagnostics to fight the pandemic". Nature - News Explainer. doi:10.1038/d41586-020-00827-6. https://www.nature.com/articles/d41586-020-00827-6. Retrieved 05 April 2020.
- ↑ Apuzzo, M.; Gebrekidan, S. (20 March 2020). "Can’t Get Tested? Maybe You’re in the Wrong Country". The New York Times. https://www.nytimes.com/2020/03/20/world/europe/coronavirus-testing-world-countries-cities-states.html. Retrieved 05 April 2020.
- ↑ Hindsley, G. (28 March 2020). "The Lost Month: How a Failure to Test Blinded the U.S. to COVID-19". The New York Times. https://www.nytimes.com/2020/03/28/us/testing-coronavirus-pandemic.html. Retrieved 05 April 2020.
- ↑ Denison, M.R. (2004). "Coronavirus Research: Keys to Diagnosis, Treatment, and Prevention of SARS". Learning from SARS: Preparing for the Next Disease Outbreak. Institute of Medicine. pp. 137–72. doi:10.17226/10915. ISBN 9780309182157. https://www.nap.edu/read/10915/chapter/5.
- ↑ Ceccarelli, M.; Berretta, M.; Venanzi Rullo, E. et al. (2020). "Differences and similarities between Severe Acute Respiratory Syndrome (SARS)-CoronaVirus (CoV) and SARS-CoV-2. Would a rose by another name smell as sweet?". European Review for Medical and Pharmacological Sciences 24 (5): 2781-2783. doi:10.26355/eurrev_202003_20551. PMID 32196628.
- ↑ Wilder-Smith, A.; Chiew, C.J.; Lee, V.J. (2020). "Can we contain the COVID-19 outbreak with the same measures as for SARS?". The Lancet Infectious Diseases. doi:10.1016/S1473-3099(20)30129-8. PMC PMC7102636. PMID 32145768. https://www.ncbi.nlm.nih.gov/pmc/articles/PMC7102636.
- ↑ World Health Organization (10 January 2020). "Laboratory testing of human suspected cases of novel coronavirus (nCoV) infection: Interim guidance 10 January 2020". WHO/2019-nCoV/laboratory/2020.1. World Health Organization. https://apps.who.int/iris/bitstream/handle/10665/330374/WHO-2019-nCoV-laboratory-2020.1-eng.pdf. Retrieved 05 April 2020.
- ↑ 50.0 50.1 50.2 50.3 World Health Organization, et al. (19 March 2020). "Laboratory testing for coronavirus disease (COVID-19) in suspected human cases: Interim guidance, 19 March 2020". WHO/COVID-19/laboratory/2020.5. World Health Organization. https://apps.who.int/iris/handle/10665/331501. Retrieved 05 April 2020.
- ↑ Terry, M. (3 April 2020). "Cellex and Mayo Clinic Launch Tests to Determine COVID-19 Immunity from Previous Exposure". BioSpace. https://www.biospace.com/article/fda-approves-1st-covid-19-antibody-test/. Retrieved 05 April 2020.
- ↑ 52.0 52.1 52.2 52.3 "Emergency Use Authorizations". U.S. Food and Drug Administration. 8 April 2020. https://www.fda.gov/medical-devices/emergency-situations-medical-devices/emergency-use-authorizations#covid19ivd. Retrieved 28 April 2020.
- ↑ 53.0 53.1 Centers for Disease Control and Prevention (24 March 2020). "Evaluating and Testing Persons for Coronavirus Disease 2019 (COVID-19)". Centers for Disease Control and Prevention. https://www.cdc.gov/coronavirus/2019-nCoV/hcp/clinical-criteria.html. Retrieved 05 April 2020.
- ↑ 54.0 54.1 Centers for Disease Control and Prevention (24 March 2020). "Interim Guidelines for Collecting, Handling, and Testing Clinical Specimens from Persons for Coronavirus Disease 2019 (COVID-19)". Centers for Disease Control and Prevention. https://www.cdc.gov/coronavirus/2019-nCoV/lab/guidelines-clinical-specimens.html. Retrieved 05 April 2020.
- ↑ 55.0 55.1 Public Health England (3 April 2020). "COVID-19: Guidance for sampling and for diagnostic laboratories". U.K Government. https://www.gov.uk/government/publications/wuhan-novel-coronavirus-guidance-for-clinical-diagnostic-laboratories. Retrieved 05 April 2020.
- ↑ 56.0 56.1 56.2 56.3 56.4 Public Health Laboratory Network (1 April 2020). "PHLN guidance on laboratory testing for SARS-CoV-2 (the virus that causes COVID-19)". Department of Health, Australian Government. https://www.health.gov.au/resources/publications/phln-guidance-on-laboratory-testing-for-sars-cov-2-the-virus-that-causes-covid-19. Retrieved 05 April 2020.
- ↑ Branswell, H. (4 April 2020). "CDC launches studies to get more precise count of undetected Covid-19 cases". STAT. https://www.statnews.com/2020/04/04/cdc-launches-studies-to-get-more-precise-count-of-undetected-covid-19-cases/. Retrieved 05 April 2020.
- ↑ 58.0 58.1 58.2 58.3 58.4 Center for Health Security (2 April 2020). "Serology-based tests for COVID-19". Johns Hopkins University. http://www.centerforhealthsecurity.org/resources/COVID-19/Serology-based-tests-for-COVID-19.html. Retrieved 05 April 2020.
- ↑ 59.0 59.1 Wang, W.; Xu, Y.; Gao, R. et al. (2020). "Detection of SARS-CoV-2 in Different Types of Clinical Specimens". JAMA. doi:10.1001/jama.2020.3786. PMC PMC7066521. PMID 32159775. https://www.ncbi.nlm.nih.gov/pmc/articles/PMC7066521.
- ↑ Yu, F.; Yan, L.; Wang, N. et al. (2020). "Quantitative Detection and Viral Load Analysis of SARS-CoV-2 in Infected Patients". Clinical Infectious Diseases: ciaa345. doi:10.1093/cid/ciaa345. PMID 32221523.
- ↑ Xu, R.; Cui, B.; Duan, X. et al. (2020). "Saliva: Potential diagnostic value and transmission of 2019-nCoV". International Journal of Oral Science 12: 11. doi:10.1038/s41368-020-0080-z.
- ↑ Greenwood, M. (24 April 2020). "Saliva samples preferable to deep nasal swabs for testing COVID-19". YaleNews. https://news.yale.edu/2020/04/24/saliva-samples-preferable-deep-nasal-swabs-testing-covid-19. Retrieved 01 May 2020.
- ↑ Vault Health (14 April 2020). "Vault Health Launches First-of-its-Kind Saliva-based FDA EUA Approved Test for COVID-19". PR Newswire. https://www.prnewswire.com/news-releases/vault-health-launches-first-of-its-kind-saliva-based-fda-eua-approved-test-for-covid-19-301039633.html. Retrieved 01 May 2020.
- ↑ 64.0 64.1 Madrigal, A.C.; Meyer, R. (31 March 2020). "Private Labs Are Fueling a New Coronavirus Testing Crisis". The Atlantic. https://www.theatlantic.com/health/archive/2020/03/next-covid-19-testing-crisis/609193/. Retrieved 07 April 2020.
- ↑ Hale, C. (17 March 2020). "FDA opens the gates to commercial coronavirus testing without agency review". FierceBiotech. https://www.fiercebiotech.com/medtech/fda-opens-gates-to-commercial-coronavirus-testing-without-agency-review. Retrieved 07 April 2020.
- ↑ 66.0 66.1 Appleby, J. (28 March 2020). "Why It Takes So Long To Get Most COVID-19 Test Results". NPR - Health Shots. https://www.npr.org/sections/health-shots/2020/03/28/822869504/why-it-takes-so-long-to-get-most-covid-19-test-results. Retrieved 07 April 2020.
- ↑ 67.0 67.1 Ryan-Mosley, T. (5 April 2020). "Why some covid-19 tests in the US take more than a week". MIT Technology Review. https://www.technologyreview.com/s/615444/covid-19-test-results-faster-commercial-labs-delays-coronavirus/. Retrieved 07 April 2020.
- ↑ Nguyen, T.; Bang, D.D.; Wolff, A. (2020). "2019 novel coronavirus disease (COVID-19): Paving the road for rapid detection and point-of-care diagnostics". Micromachines 11 (3): 306. doi:10.3390/mi11030306. PMID 32183357.
- ↑ Yang, T.; Wang, Y.-C.; Shen, C.-F.; Cheng, C.-M. (2020). "Point-of-care RNA-based diagnostic device for COVID-19". Diagnostics 10 (3): 165. doi:10.3390/diagnostics10030165.
- ↑ 70.0 70.1 Centers for Disease Control and Prevention (6 August 2018). "Clinical Laboratory Improvement Amendments (CLIA): Test complexities". https://www.cdc.gov/clia/test-complexities.html. Retrieved 09 April 2020.
- ↑ "CLIA Categorizations". U.S. Food and Drug Administration. 25 February 2020. https://www.fda.gov/medical-devices/ivd-regulatory-assistance/clia-categorizations. Retrieved 09 April 2020.
- ↑ 72.0 72.1 72.2 Centers for Medicare and Medicaid Services, Division of Clinical Laboratory and Quality (October 2019). "CLIA Update - October 2019" (PDF). https://www.cms.gov/Regulations-and-Guidance/Legislation/CLIA/Downloads/statupda.pdf. Retrieved 09 April 2020.
- ↑ Garrels, M.; Oatis, C.S. (2014). Laboratory and Diagnostic Testing in Ambulatory Care: A Guide for Healthcare Professionals (3rd ed.). Elsevier Health Sciences. pp. 368. ISBN 9780323292368. https://books.google.com/books?id=LM9sBQAAQBAJ. Retrieved 09 April 2020.
- ↑ U.S. Food and Drug Administration (10 April 2020). "FAQs on Diagnostic Testing for SARS-CoV-2". U.S. Food and Drug Administration. https://www.fda.gov/medical-devices/emergency-situations-medical-devices/faqs-diagnostic-testing-sars-cov-2. Retrieved 10 April 2020.
- ↑ Rakeman, J.L. (7 April 2020). "COVID-19 Serology Testing" (PDF). New York City Department of Health and Mental Hygiene. https://www1.nyc.gov/assets/doh/downloads/pdf/imm/covid-19-serology-testing.pdf. Retrieved 09 April 2020.
- ↑ Simonite, T. (24 March 2020). "The Professors Who Call ‘Bullshit’ on Covid-19 Misinformation". Wired. https://www.wired.com/story/professors-call-bullshit-covid-19-misinformation/. Retrieved 09 April 2020.
- ↑ Suciu, P. (8 April 2020). "During COVID-19 Pandemic It Isn't Just Fake News But Seriously Bad Misinformation That Is Spreading On Social Media". Forbes. https://www.forbes.com/sites/petersuciu/2020/04/08/during-covid-19-pandemic-it-isnt-just-fake-news-but-seriously-bad-misinformation-that-is-spreading-on-social-media/. Retrieved 09 April 2020.
- ↑ 78.0 78.1 Foundation for Innovative New Diagnostics (27 November 2024). "SARS-CoV-2 Diagnostic Pipeline". Foundation for Innovative New Diagnostics. https://www.finddx.org/covid-19/pipeline/. Retrieved 10 April 2020.
- ↑ 79.0 79.1 Bistricean, C. (1 April 2020). "COVID-19 Testing for the Physician Office Laboratory (POL) - CovidLiMS.com". Medium. https://medium.com/@lablynx/covid-19-testing-for-the-physician-office-laboratory-pol-covidlims-com-bf1615e071ab. Retrieved 10 April 2020.
- ↑ Dickens, J.F. (3 April 2020). "Coronavirus testing: How it works – Questions answered". Reaction. https://reaction.life/coronavirus-testing-how-it-works-questions-answered/. Retrieved 10 April 2020.
- ↑ Hinton, D.M. (27 March 2020). "ID NOW COVID-19". U.S. Food and Drug Administration. https://www.fda.gov/media/136522/download. Retrieved 10 April 2020.
- ↑ 82.0 82.1 Schmid-Burgk, J.L.; Li, D.; Feldman, D. et al. (2020). "LAMP-Seq: Population-Scale COVID-19 Diagnostics Using a Compressed Barcode Space". bioRxiv. doi:10.1101/2020.04.06.025635.
- ↑ Lamb, L.E.; Barolone, S.N.; Ward, E. et al. (2020). "Rapid Detection of Novel Coronavirus (COVID-19) by Reverse Transcription-Loop-Mediated Isothermal Amplification". medRxiv. doi:10.1101/2020.02.19.20025155.
- ↑ Yu, L.; Wu, S.; Hao, X. et al. (2020). "Rapid colorimetric detection of COVID-19 coronavirus using a reverse tran-scriptional loop-mediated isothermal amplification (RT-LAMP) diagnostic plat-form: iLACO". medRxiv. doi:10.1101/2020.02.20.20025874.
- ↑ Park, G.-S.; Ku, K.; Baek, S.-H. et al. (2020). "Development of Reverse Transcription Loop-mediated Isothermal Amplification (RT-LAMP) Assays Targeting SARS-CoV-2". bioRxiv. doi:10.1101/2020.03.09.983064.
- ↑ "Detect COVID-19 in as Little as 5 Minutes". Abbott. 27 March 2020. https://www.abbott.com/corpnewsroom/product-and-innovation/detect-covid-19-in-as-little-as-5-minutes.html. Retrieved 10 April 2020.
- ↑ "A protocol for rapid detection of the 2019 novel coronavirus SARS-CoV-2 using CRISPR diagnostics: SARS-CoV-2 DETECTR" (PDF). Mammoth Biosciences. 2 March 2020. https://mammoth.bio/wp-content/uploads/2020/03/Mammoth-Biosciences-A-protocol-for-rapid-detection-of-SARS-CoV-2-using-CRISPR-diagnostics-DETECTR.pdf. Retrieved 09 April 2020.
- ↑ "CRISPR’s powers unleashed for disease detection". Nature - Research Highlights. 16 February 2018. https://www.nature.com/articles/d41586-018-02200-0. Retrieved 09 April 2020.
- ↑ 89.0 89.1 Terry, M. (30 March 2020). "CRISPR Pioneer Jennifer Doudna Expects Automated COVID-19 Test Lab to Begin Testing Next Week". BioSpace. https://www.biospace.com/article/crispr-discoverer-jennifer-doudna-launches-covid-19-test-lab/. Retrieved 10 April 2020.
- ↑ 90.0 90.1 Sands, P.; Mundaca-Shah, C.; Dzau, V.J. (2016). "The Neglected Dimension of Global Security — A Framework for Countering Infectious-Disease Crises". The New England Journal of Medicine 374 (13): 1281–87. doi:10.1056/NEJMsr1600236. PMID 26761419.
- ↑ Morris, C. (26 February 2020). "Trump administration budget cuts could become a major problem as coronavirus spreads". Fortune. https://fortune.com/2020/02/26/coronavirus-covid-19-cdc-budget-cuts-us-trump/. Retrieved 10 April 2020.
- ↑ Specter, M. (17 March 2020). "The Coronavirus and the Gutting of America’s Public-Health System". The New Yorker. https://www.newyorker.com/news/daily-comment/coronavirus-and-the-gutting-of-americas-public-health-system. Retrieved 10 April 2020.
- ↑ Roos, R. (25 May 2007). "Congress cut pandemic funds before passing spending bill". CIDRAP - News & Perspective. https://www.cidrap.umn.edu/news-perspective/2007/05/congress-cut-pandemic-funds-passing-spending-bill. Retrieved 10 April 2020.
- ↑ Schnirring, L. (20 December 2007). "Congress slashes pandemic preparedness funding". CIDRAP - News & Perspective. https://www.cidrap.umn.edu/news-perspective/2007/12/congress-slashes-pandemic-preparedness-funding. Retrieved 10 April 2020.
- ↑ Gates, B. (2020). "Responding to Covid-19 — A Once-in-a-Century Pandemic?". The New England Journal of Medicine. doi:10.1056/NEJMp2003762. PMID 32109012.
- ↑ Shontell, A. (10 April 2020). "Melinda Gates: This is not a once-in-a-century pandemic. 'We will absolutely have more of these.' The billionaire philanthropist predicts a timeline for going back to normal". Business Insider. https://www.businessinsider.com/melinda-gates-coronavirus-interview-vaccine-timeline-2020-4. Retrieved 10 April 2020.
- ↑ Madhav, N.; Oppenheim, B.; Gallivan, M. et al. (2017). "Chapter 17: Pandemics: Risks, Impacts, and Mitigation". In Jamison, D.T.; Gelband, H.; Horton, S. et al.. Disease Control Priorities: Improving Health and Reducing Poverty (3rd ed.). The World Bank. ISBN 9781464805288. https://www.ncbi.nlm.nih.gov/books/NBK525302/.
- ↑ Sanders, R. (30 March 2020). "UC Berkeley scientists spin up a robotic COVID-19 testing lab". Berkeley News. https://news.berkeley.edu/2020/03/30/uc-berkeley-scientists-spin-up-a-robotic-covid-19-testing-lab/. Retrieved 10 April 2020.
- ↑ 99.0 99.1 Nolting, R. (10 April 2020). "Local testing delayed by lack of reagents". Parsons Sun. https://www.parsonssun.com/news/coronavirus/article_99193646-7aec-11ea-a7c5-b700f82332db.html. Retrieved 10 April 2020.
- ↑ Herper, M.; Branswell, H. (10 March 2020). "Shortage of crucial chemicals creates new obstacle to U.S. coronavirus testing". STAT. https://www.statnews.com/2020/03/10/shortage-crucial-chemicals-us-coronavirus-testing/. Retrieved 10 April 2020.
- ↑ Hale, C. (18 March 2020). "Qiagen aims to more than quadruple its COVID-19 reagent production in 6 weeks". Fierce Biotech. https://www.fiercebiotech.com/medtech/qiagen-aims-to-more-than-quadruple-its-covid-19-reagent-production-6-weeks. Retrieved 10 April 2020.
- ↑ 102.0 102.1 Mehta, A. (3 April 2020). "Mystery surrounds UK claim of Covid-19 test reagent ‘shortage’". Chemistry World. https://www.chemistryworld.com/mystery-surrounds-uk-claim-of-covid-19-test-reagent-shortage/4011457.article. Retrieved 10 April 2020.
- ↑ 103.0 103.1 Roche, B. (8 April 2020). "Irish scientists develop reagent in effort to ease Covid-19 testing delays". The Irish Times. https://www.irishtimes.com/news/science/irish-scientists-develop-reagent-in-effort-to-ease-covid-19-testing-delays-1.4223897. Retrieved 10 April 2020.